During photosynthesis, a symphony of chemicals transforms light into the energy required for plant, algal, and some bacterial life. Scientists now know that this remarkable reaction requires the smallest possible amount of light – just one single photon – to begin.
A US team of researchers in quantum optics and biology showed that a lone photon can start photosynthesis in the purple bacterium Rhodobacter sphaeroides, and they are confident it works in plants and algae since all photosynthetic organisms share an evolutionary ancestor and similar processes.
The team says their findings bolster our knowledge of photosynthesis and will lead to a better understanding of the intersection of quantum physics in a wide range of complex biological, chemical, and physical systems, including renewable fuels.
"A huge amount of work, theoretically and experimentally, has been done around the world trying to understand what happens after a photon is absorbed," says Graham Fleming, a biochemist at the University of California, Berkeley.
"But we realized that nobody was talking about the first step. That was still a question that needed to be answered in detail."
Chlorophyll molecules receive photons from the Sun, where the chlorophyll's electron becomes excited, hopping over to different molecules to form sugar's building blocks, giving plants food and releasing oxygen.
The Sun doesn't shower us with an overly generous number of photons – on a sunny day, only around 1,000 photons reach a chlorophyll molecule each second – so the efficiency of photosynthesis in harnessing sunlight to produce energy-rich molecules led scientists to believe that a single photon could start this reaction.
"Nature invented a very clever trick," Fleming says.
The researchers focused on a well-studied structure of proteins in purple bacteria, called the light-harvesting 2 (LH2) complex, that can absorb photons at a particular wavelength.
Using specialized tools, they created a photon source that made a pair of photons from one photon of higher energy using spontaneous parametric down-conversion.
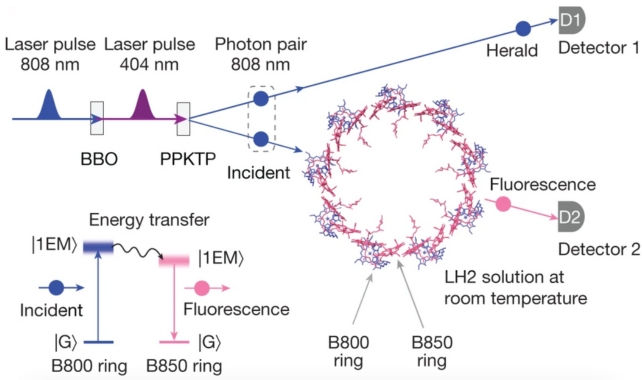
During a pulse, the first photon, called "the herald," was observed with a highly sensitive detector, signaling its partner photon's arrival, which interacted with LH2 molecules in a laboratory sample of the purple bacteria.
When a photon with a wavelength of 800 nanometers hit a ring of molecules in LH2, the energy went to a second ring, which gave off fluorescent photons with a wavelength of 850 nanometers.
In nature, this energy transfer would continue until it started the process of photosynthesis. Finding a photon with a wavelength of 850 nanometers in the laboratory was a clear sign that that process had begun, especially as the LH2 structures were separated from other parts of the cell.
The challenge was dealing with single photons, which are easy to lose. To get around this, the scientists used the herald photon as a guide.
"I think the first thing is that this experiment has shown that you can actually do things with individual photons," says chemical physicist Birgitta Whaley from Berkeley. "So that's a very, very important point."
Using a probability distribution model and a computer algorithm, the team analyzed over 17.7 billion herald photon detection events and 1.6 million fluorescent photon detection events.
The thorough analysis means the researchers are confident the results were only caused by single-photon absorption and no other factors could have had an effect.
Much of the prior research done on the later, post-light absorption steps of photosynthesis has involved sending powerful, ultra-fast laser pulses to photosynthetic molecules.
"There's a huge difference in intensity between a laser and sunlight – a typical focused laser beam is a million times brighter than sunlight," explains Quanwei Li, a quantum physicist and engineer from Berkeley.
By demonstrating how individual photons behave during photosynthesis, this research gives us important information about how nature's energy conversion process works. Artificial photosynthesis techniques may one day be the key to sustainably surviving and thriving in space.
"Just like you need to understand each particle to build a quantum computer," Li adds, "we need to study the quantum properties of living systems to truly understand them and to make efficient artificial systems that generate renewable fuels."
This study was a unique opportunity for two scientific fields that don't usually work together to apply and combine the techniques of quantum optics and biology.
"The next thing is, what else can we do?" says Whaley.
"Our goal is to study the energy transfer from individual photons through the photosynthetic complex at the shortest possible temporal and spatial scales."
The research has been published in Nature.