More than sixty years ago, the Search for Extraterrestrial Intelligence (SETI) officially began with Project Ozma at the Greenbank Observatory in West Bank, Virginia.
Led by famed astronomer Frank Drake (who coined the Drake Equation), this survey used the observatory's 25-meter (82-foot) dish to monitor Epsilon Eridani and Tau Ceti – two nearby Sun-like stars – between April and July of 1960.
Since then, multiple surveys have been conducted at different wavelengths to search for indications of technological activity (aka. 'technosignatures') around other stars.
While no conclusive evidence has been found that indicates the presence of an advanced civilization, there have been many cases where scientists could not rule out the possibility.
In a recent paper, veteran NASA scientist Richard H. Stanton describes the results of his multi-year survey of more than 1300 Sun-like stars for optical SETI signals. As he indicates, this survey revealed two fast identical pulses from a Sun-like star about 100 light-years from Earth, that match similar pulses from a different star observed four years ago.
Dr. Stanton is a veteran of NASA's Jet Propulsion Laboratory (JPL), whose work includes participating in the Voyager missions and serving as the Engineering Manager of the Gravity Recovery And Climate Experiment (GRACE) mission.
Since retiring, he has dedicated himself to the Search for Extraterrestrial Intelligence (SETI) using the 76.2-cm (30-inch) telescope at the Shay Meadow Observatory in Big Bear, California, and a multi-channel photometer he designed. The paper describing his survey's findings appeared in the journal Acta Astronautica.
For years, Stanton has used these instruments to observe more than 1,300 Sun-like stars for optical SETI signals. Unlike traditional SETI surveys that have used radio antennas to search for evidence of potential extraterrestrial transmissions, optical SETI looks for pulses of light that could result from laser communications or directed-energy arrays.
This latter example has been considered in recent years thanks to Project Starshot, NASA's Directed Energy Propulsion for Interstellar Exploration (DEEP-IN) concept, and similar interstellar mission concepts.
As Stanton indicated, the field of optical SETI traces its roots to a 1961 study by Schwartz and Townes. They reasoned that the best way an extraterrestrial intelligence (ETI) could send an optical signal that outshone their star would be with intense nanosecond laser pulses.
These pulses are searched for using special equipment in infrared wavelengths, high-resolution spectra, or visible light. As Stanton related to Universe Today via email, his SETI search differs from conventional optical surveys:
"My approach is to stare at a single star for roughly 1 hour using photon counting to sample the star's light at what is considered a very high time-resolution for astronomy (100 microsecond samples).
"The resulting time series are then searched for pulses and optical tones. The instrument uses readily available off-the-shelf components that can be assembled into a PC-based system. I'm not sure if anyone else is doing this with a significant time commitment. I am not aware of any discovery of similar pulses."
After years of searching, Stanton reported an unexpected "signal" when observing HD 89389, an F-type star slightly brighter and more massive than our Sun, located in the constellation Ursa Major.
According to Stanton's paper, this signal consisted of two fast, identical pulses 4.4 seconds apart that were not revealed in previous searches. He then ran comparisons against signals produced by airplanes, satellites, meteors, lightning, atmospheric scintillation, system noise, etc.
As he explained, several things about the pulses detected around HD89389 made them unique from anything seen previously:
"a. The star gets brighter-fainter-brighter and then returns to its ambient level, all in about 0.2s. This variation is much too strong to be caused by random noise or atmospheric turbulence. How do you make a star, over a million kilometers across, partially disappear in a tenth of a second? The source of this variation can't be as far away as the star itself.
b. In all three events, two essentially identical pulses are seen, separated by between 1.2 and 4.4 seconds (the third event, found in an observation on January 18th of this year, was not included in the paper). In over 1500 hours of searching, no single pulse resembling these has ever been detected.
c. The fine structure in the star's light between the peaks of the first pulse repeats almost exactly in the second pulse 4.4s later. No one knows how to explain this behavior.
d. Nothing was detected moving near the star in simultaneous photography or in the background sensor that easily detects distant satellites moving close to a target star. Common signals from airplanes, satellites, meteors, birds, etc., are completely different from these pulses."
A re-examination of historical data for similar signals revealed another pair of pulses detected around HD 217014 (51 Pegasi) in 2021. This main-sequence G-type star is located about 50.6 light-years from Earth and is similar in size, mass, and age to our Sun.
In 1995, astronomers at the Observatoire de Haute-Provence detected an exoplanet orbiting this star, a hot gas giant that has since been named Dimidium. This was one of the first exoplanets ever detected, and the first time an exoplanet was discovered around a main-sequence star.
At the time, said Stanton, the signal was dismissed as a false positive caused by birds. However, a detailed analysis ruled out this possibility for all the pulses observed. Other possibilities that Stanton explores include diffraction caused by the Earth's atmosphere, possibly due to a shock wave.
However, this is unlikely since shockwaves would have had to occur with perfect timing to coincide with both optical pulses. Other possibilities include starlight diffraction by a distant body in the Solar System, partial eclipses caused by Earth satellites or distant asteroids, and 'edge diffraction' by a straight edge (as described by the Sommerfeld Effect).
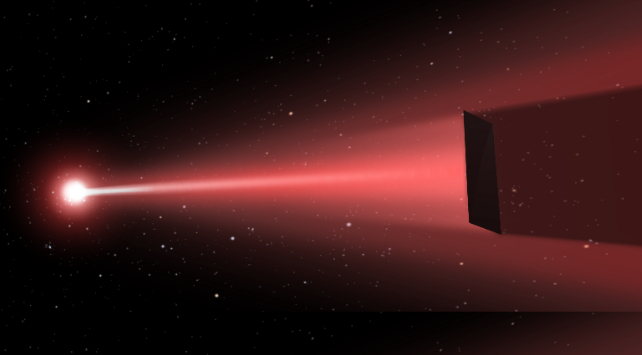
There's also the possibility that a gravity wave could have generated these pulses, which requires additional consideration. Another interesting possibility is that it could be the result of ETI.
As Stanton indicated, whatever modulated these stars' light must be relatively close to Earth, implying that any ETI activity must be within our Solar System. However, Stanton stresses that more data is needed.
"None of these explanations are really satisfying at this point," he said. "We don't know what kind of object could produce these pulses or how far away it is. We don't know if the two-pulse signal is produced by something passing between us and the star or if it is generated by something that modulates the star's light without moving across the field. Until we learn more, we can't even say whether or not extraterrestrials are involved!"
There are several examples of Optical SETI (OSETI) or LaserSETI, including the collaborative effort launched by Breakthrough Listen and the Very Energetic Radiation Imaging Telescope Array System (VERITAS) Collaboration.
However, Stanton's method presents many opportunities for future SETI surveys, which could search for similar examples of optical pulses. To that end, he suggests two approaches that could reveal more about this phenomenon and help astronomers place tighter constraints on their possible causes:
"Look for events using arrays of synchronized optical telescopes. If the object is moving between the star and us, this approach should tell us how fast it is moving normal to the line of sight, and potentially its size and distance.
"[Also,] it would be very interesting if the star's light is modulated without an object moving across the field. Observing events with telescopes separated by a few hundred kilometers might show that any separation in the time each pulse arrives is due only to differences in the light time from the star to each telescope. Then, unless the variation could somehow be attributed to the star itself, we would have even more to explain!"
This article was originally published by Universe Today. Read the original article.