As far as gravity is concerned, both matter and its charge-flipped 'mirror' twin, antimatter, deserve a big, warm hug.
A recent experiment by international Antihydrogen Laser Physics Apparatus (ALPHA) collaboration achieved the first direct measurement of free-falling antimatter.
Had the results been any different, we might have at last had our first solid clue on where half of the Universe went some 13.4 billion years ago.
Antimatter is the quantum shadow of the familiar kind of matter that makes up objects in our everyday lives.
Reverse the charge (and a handful of other more subtle characteristics) of an electron, you get an equally tiny, yet positively charged particle called a positron.
Build a proton out of antiquarks, you get a negative equivalent called an antiproton.
And if you put the two together? You get an atom of antihydrogen, with a positive particle orbiting a negative nucleus.
The Universe isn't shy in creating antimatter particles, at least no more than it is in creating matter. But bring the two together and their opposing flavors will quickly cancel out, leaving nothing but a rapidly expanding bubble of gamma rays to suggest they ever existed.
This blunt fact of cosmic bookkeeping poses a mystery – why do we have a Universe filled with matter-stars, matter-planets, and matter-galaxies, if an equivalent amount of antimatter appeared in the raging furnace of the Big Bang immediately to cancel it all out?
While some physicists have questioned premises of antimatter creation and cancellation, others have challenged assumptions of the mirror twin's fundamental behaviors.
For example, if positrons were pushed away by gravity rather than pulled, the two kinds of matter could have been divided early in the Universe's creation.
It's a neat idea, even if it isn't a concept supported by theory.
"If antihydrogen were somehow different from hydrogen, that would be a revolutionary thing because the physical laws, both in quantum mechanics and gravity, say the behavior should be the same," says University of California, Berkeley plasma physicist and ALPHA collaboration member Jonathan Wurtele.
"However, one doesn't know until one does the experiment."
Conducting such an experiment is easier said than done.
We've been comparing gravity's influence on different kinds of matter under what's known as the weak equivalence principle long before Galileo allegedly dropped ammunition from the top of the tower at Pisa.
Dropping a mass made of antimatter is a whole other ballgame.
For one thing, while we can synthesize it using a particle collider, barely a few nanograms of antiprotons and positrons have been produced in all of history.
Slowing antimatter down, storing it, and transporting the antiparticles for experimentation is problem number two.
Perhaps the biggest hurdle, however, is gravity's own incredible weakness compared with the incredible might of electromagnetism. The force of gravity on a single proton sitting on Earth's surface is the same as an electric field of just 0.000001 volts per square meter.
Magnetic fields are even more disastrous, requiring experimentalists to think well outside of the box in devising ways to see whether an antimatter object falls like one made of matter.
In 2022, researchers found indirect evidence that the two responded to gravity the same way. By racing the two kinds of matter around a trap over the course of 18 months, they found no sign that gravity was having a different effect on antimatter.
To take experimentation one step further, ALPHA devised a vertical vacuum chamber with adjustable magnetic fields and 'dropped' particles of antihydrogen down its center.
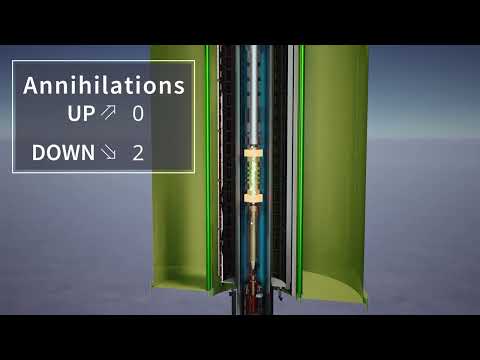
"Broadly speaking, we're making antimatter and we're doing a Leaning Tower of Pisa kind of experiment," says Wurtele.
By varying the magnetic fields in a controlled fashion, the researchers could cancel out their effects, leaving the pull of gravity to bias more particles into falling Earthward than accelerating skyward.
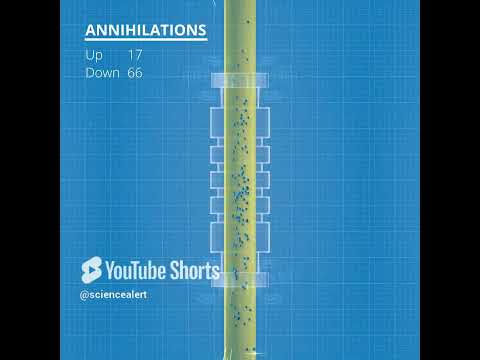
The experiment means we can put aside suggestions that gravity sifted antimatter out of the ashes of the Big Bang, at least for now.
Yet there is still a slim possibility that gravity loves one of matter's twins a little more than the other. If it does, it might yet explain why we inherited a whole Universe.
This research was published in Nature.