In an ongoing quest to guess the secret behind the Universe's excess in gravity, two researchers from Dartmouth College in the US have proposed a chilling union between massless particles soon after the Big Bang.
For the better part of a century it's been frustratingly clear that estimates of the Universe's visible mass have failed to account for the way galaxies rotate, pointing to slow-moving clumps of matter we can't see. This stuff has been dubbed ' dark matter'.
Even as researchers whittle away at the list of properties describing this cold and silent corner of physics, its identity and origins remain elusive.
Physicists Guanming Liang and Robert Caldwell picture a newborn cosmos sizzling with massless particles zooming about at high speed – a form of matter that has more in common with light than with cold chunks of darkness.
Over time, particles within this fog of high-energy material collided and cooled, leaving them with the required mass to explain the Universe's unseen source of gravity.
"That's totally antithetical to what dark matter is thought to be – it is cold lumps that give galaxies their mass," Caldwell says.
"Our theory tries to explain how it went from being light to being lumps."
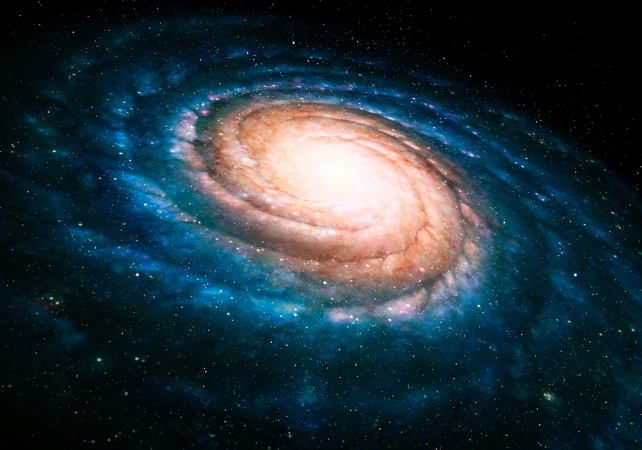
Roughly 13.7 billion years ago, when the whole Universe was crammed into a space that would easily fit in a gap in your teeth, a quantum party was underway. Particles of all varieties were zooming about, bumping and grinding for want of room to move.
According to the Nambu and Jona-Lasinio model of nucleon synthesis, one particular class of these particles known as Dirac fermions could buddy up in a very similar manner to the way electrons combine to form Cooper pairs in superconductors.
Though the physics behind the activity is complex (even for quantum mechanics), there are implications for cosmological growth, from cosmic inflation to later expansion. Yet all have been based on assumptions that the temperature of this space remained fairly balanced.
Liang and Caldwell wondered what might happen if other thermal properties of this process were taken into account. What if an imbalance in the partnerships between some hypothetical high-energy Dirac fermions resulted in them converting their insane energy to mass, effectively freezing them?
The product would be like turning a thundercloud into a hailstorm.
"The most unexpected part of our mathematical model was the energy plummet that bridges the high-density energy and the lumpy low energy," says Liang.
The very fact Cooper pairs exist among electrons means no exotic activity is required to explain the emergence of these slow, dark matter particles. What's more, the hypothesis could explain where so much of the energy in the early Universe went.
"Structures get their mass due to the density of cold dark matter, but there also has to be a mechanism wherein energy density drops to close to what we see today," Liang says.
"The mathematical model of our theory is really beautiful because it's rather simplistic – you don't need to build a lot of things into the system for it to work."
Being simple is one thing. Proving it is another. Unlike so many proposals on the identity of the mysterious material known as dark matter, this theory could actually be tested using data we already have at hand.
Transformations from hot, high-pressure loners to cold and slow marriages would leave a signature in the cosmic microwave background – the distorted background glow of radiation that's been bouncing around the Universe since its earliest moments.
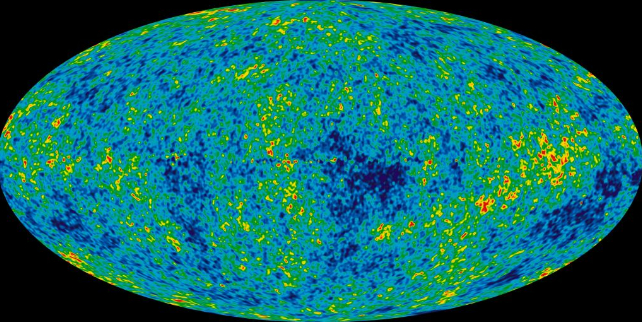
Certain signs found in the cosmic microwave background would be points scored in favor of these fermions being at least one source of dark matter.
"It's exciting," says Caldwell. "We're presenting a new approach to thinking about and possibly identifying dark matter."
This research was published in Physical Review Letters.