Life is not always straightforward about the business of living.
A food web, for instance, consists of chains upon chains of organisms stealing other organisms' energy-processing abilities.
We can't process sunlight into the sugars we need for energy; we wait for plants to do it, and eat them. Carnivores can't properly digest plants; they wait for other animals to eat plants, then eat those animals.
In other words, most of us piggyback off other organisms in some way – but parasites take the notion to its ultimate conclusion. Why go about the hard work of living when you can get some other creature to do it for you? Or, even better, make them do it for you.
This is the wild west of parasitology: when a parasite doesn't just hitch a ride, but hijacks the body of their host in some way, driving it to behaviors that benefit the parasite – often to their own great, even fatal, detriment.
"In the scientific literature, the name for that is adaptive host manipulation hypothesis," parasitologist Alex Maier of the Australian National University told ScienceAlert.
"That means that the parasites actually induce modification in the host, that could maximize the parasite sickness. So that the parasite is distributed more often, and there are more parasites out there."
You may have heard of this before. One of the most well-known examples is Toxoplasma gondii, which causes a disease called toxoplasmosis.
Studies have found that animals infected with T. gondii exhibit behavioral changes, taking more risks, and behaving in ways that may make them more likely to encounter cats – the only host in which the parasite can reproduce.
Whether or not T. gondii does actually manipulate hosts is still under investigation, but T. gondii is just one parasite. There are many parasites out there tweaking their hosts in different ways… and the fascinating thing is that often we have no idea how they actually do it.
Let's get physical
Parasites live a very complicated and circuitous life, but it seems to be incredibly effective. While some parasites stick to one host for their whole life cycle, others require multiple hosts to complete their biological journeys.
Here's how the more complex life cycles tend to work. A parasite reproduces and lays eggs in what is known as the definitive host. Those eggs are shed into the environment, usually in feces; the eggs or larvae are then taken up by the first intermediate host.
There, the parasite continues to develop, and from here can either continue to grow to maturity, or make its way to a second intermediate host to complete its growth.
Once it is fully grown and ready to reproduce, it needs to make its way back to the definitive host – often a specific type of animal, like T. gondii and cats.
Which is where adaptive host manipulation comes into play.
The most obvious way a parasite can manipulate a host is by changing its body in some way. Some of these are spectacularly weird.
Ribeiroia ondatrae, the frog-mutating flatworm, is a flatworm that, like its name indicates, mutates frogs. Specifically, it seems to target the frogs' hind legs. Frogs infected with this parasite grow additional, useless limbs – sometimes lots of them.
Another flatworm, called Leucochloridium paradoxum (the green-banded broodsac), enters the eyestalks of snails, where it swells and pulsates, creating an eye-catching display of movement and color.
In both of these cases, the ultimate target of the parasites is not the frogs, or the snails. Both R. ondatrae and L. paradoxum live and grow in the bodies of their intermediate hosts, but they can only reproduce in the bodies of birds (or also mammals for R. ondatrae).
Their ultimate aim, therefore, is increasing the likelihood that the frogs and snails (or their eyestalks, anyway) will get eaten up, taking the parasites with them.
In the case of the frogs, the parasite reduces its mobility, and ability to flee. In the case of the snails, the colorful display is like a neon sign saying to birds "SNACKS THIS WAY". (Interestingly, Maier notes, the snails' eyestalks regenerate after being eaten by a bird, so the same snail can provide many snacks for birds.)
In these cases, researchers have an understanding of how the parasites are doing what they do.
The larvae of R. ontradae are attracted to the limb buds of their second intermediate amphibian hosts; they encyst themselves around this region, where they influence the growth of limbs. L. paradoxum puts itself where it will be most visible and attractive to predators.
It's not always that simple though.
It's all in the mind
L. paradoxum has another trick up its sleeve, and this one is a little more difficult to figure out. Snails infected with the parasite also behave differently from uninfected snails.
"Green banded broodsac also alter their behavior, not only the morphology," Maier explains.
"So that's the swelling of the eyestalks and this pulsating behavior in the eyestalks. Similarly, they move out into the open so that the birds come in, and then they can pick off those eye stalks."
A whole plethora of parasite species have been linked with similar behaviors. Pomphorhynchus laevis, the thorny-headed worm, inverts its shrimp intermediate host's reaction to light, which makes them more vulnerable to predation by the carp that are the definitive host.
Cane toads infected with the lungworm Rhabdias pseudosphaerocephala seek out warmer temperatures and moister environments, which increase the growth and survivability of the parasites, respectively.
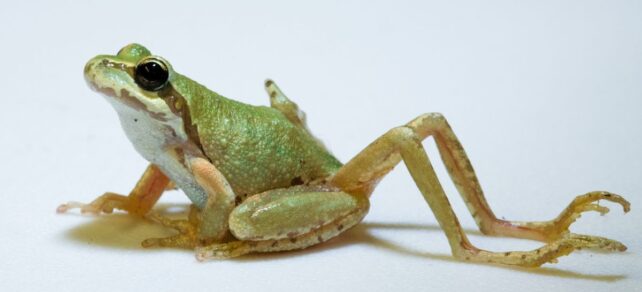
One particularly fascinating example is Dicrocoelium dendriticum, the lancet liver fluke, which targets sheep and cattle as its definitive host – but how it gets to sheep and cattle is truly devious.
Once the fluke has reproduced, the eggs are shed with the feces of the host, and subsequently eaten by snails, where they hatch and develop. At this point, they make their exit through the snails' slime, which contains a sugary substance that is very delicious to ants.
Inside the ant, the parasite develops to maturity… and from there, the ant is eaten by cattle and sheep, where it can begin the life cycle all over again.
"The logical flaw is the cow is vegetarian. Why should they eat ants?" Maier says.
"Actually, infected ants climb up grass in the evenings. During the day they show normal behavior. In the evenings when the temperature drops, normally the ants go back into their nest. But those infected ones climb up the grass. And then when the temperature drops further, they spasm in their mandibles, and they actually lock themself to that grass.
"So they spent the night on that grass; in the morning the cow comes along, eats the grass, and picks up those infected ants."
Somehow, the parasite hijacks the ant and drives it to fatal behavior to get back to the fluke sex party happening in cows.
Or is it in the mind?
We do know, a little, about how D. dendriticum might be pulling this off. A few years ago, a team of researchers conducted CT scans of parasitized ants, and found that, while the majority of the parasites were clustered in the abdomen, there was almost always one lone parasite situated right next to the ant's brain.
We still don't know what exactly that pilot fluke does, but it could be manipulating the ant's brain, either chemically or physically.
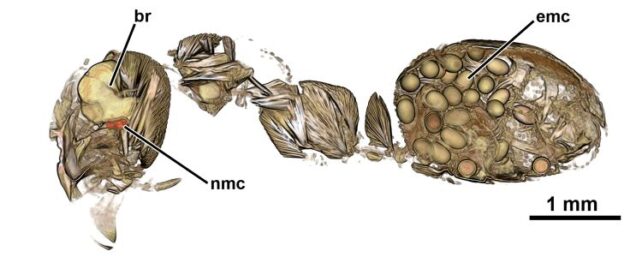
In many cases, though, we don't know the actual strings being pulled, especially for human parasites. The reason for this is that we're usually relying on behavioral data, and behavior is a complex thing with countless possible drivers, including personality. And there can be bias in the way we select behavioral data for inclusion in research.
A birth-cohort study published in 2016, for example, found little evidence to support the notion that Toxoplasma gondii leads to increased risk of psychiatric disorders or increased impulsivity in humans, as had previously been asserted.
In fact, it's not definitively clear that T. gondii manipulates rodents, either. A 2013 study found the evidence unconvincing. It can be extremely difficult to conclusively link such changes to parasite manipulation.
Perhaps the host is simply behaving differently because they feel ill… you know, because they have a parasitic infection.
The case for rabies is more compelling. Behavioral symptoms include hyperactivity, insomnia, and an extreme fear of water and fresh air, which scientists have linked to a toxin-like neuroinhibitor released by the virus Rabies lyssavirus.
By the time symptoms appear in rabies, it's too late to do anything about it; such cases are almost always fatal. And we have no way to diagnose rabies prior to symptom onset.
Although relatively few parasites are known to alter human behavior, though, adaptive host manipulation is a field that warrants further investigation. These tiny hitch-hikers actually have a huge impact on the larger world.
Driving evolution
The battle between parasite and host is a sort of evolutionary arms race. The parasite wants to maximize its chances of arriving at its definitive host; the host wants to minimize its chances of parasite sickness.
So parasites will develop adaptations that help it infect its hosts, and the hosts increase resistance and tolerance to the parasites in response.
This can have some pretty interesting consequences. One psychologist even theoretically explored the notion that the evolution of the human brain could have, at least in part, been driven by parasites.
While purely speculative, the idea has some interesting implications, such as mimicking a parasite attack to stimulate the brain's defense mechanisms.
It's not that strange an idea. Recent research has shown promise in treating irritable bowel conditions such as coeliac disease and Crohn's disease with parasitic worms that live in the gut, known as helminth therapy.
Patients with coeliac disease showed a massive increase in gluten tolerance after being infected with hookworms, and Crohn's disease patients treated with whipworms often enter remission.
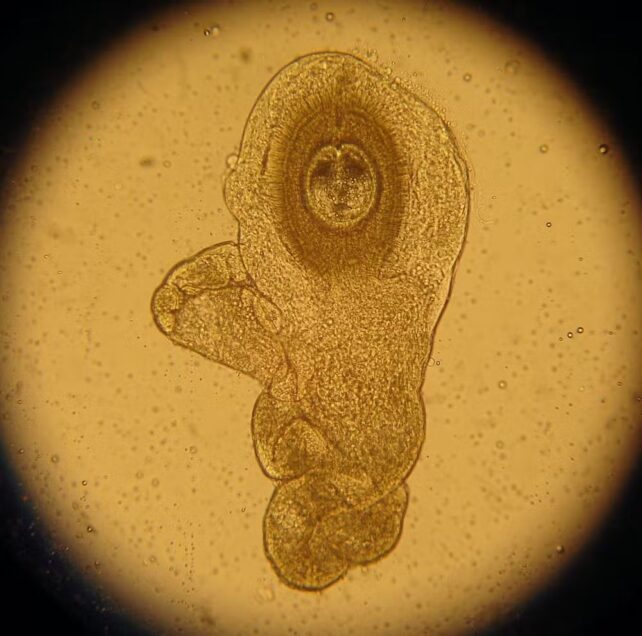
These are autoimmune conditions, and the response to parasite infection suggests one potential reason for it, Maier says.
"In the last 50 years, some parts of the human race have grown up in a hygienic environment – that's the hygiene hypothesis," he explains.
"And the part of our immune system that normally used to deal with those wormy parasites is now sitting idle. It has nothing to do. And in some individuals, it starts attacking their own tissue."
Parasitism forces both hosts and parasites to diversify, to enter new ecological niches, to adapt. Populations of animals with parasites have greater genetic diversity, because that limits parasite success.
If we can link parasite behavior to our own evolutionary adaptations, that could have some implications for how we treat, or even prevent, some forms of disease.
Beyond that, however, parasites that are able to manipulate their hosts give us clues to understanding their biology. Altering a neurotransmitter, for example, as rabies does, helps us learn how that neurotransmitter works.
We have a tendency to think of parasites with disgust and revulsion, but there's a lot more going on with these mooching organisms than pulsating eyestalks, no matter how vivid and eye-catching they are.
Maybe the idea of adaptive host manipulation opens up all sorts of philosophical questions about the malleability of our own identities, but perhaps our tiny passengers are also driving us all onward and upward to greater things.