Electrons are marvelous little things. They often hang about orbiting atomic nuclei, but they don't have to – the Universe is full of loose electrons rattling about.
Ninety years ago, theoretical physicist Eugene Wigner proposed that they didn't have to rattle about, either: that free electrons may be forced together in a peculiar kind of matter that has no atoms at all, just electrons trapped by their own repulsion a neat, crystalline lattice.
This is known as a Wigner crystal, and physicists have finally obtained direct observational evidence that it can exist.
"The Wigner crystal is one of the most fascinating quantum phases of matter that has been predicted and the subject of numerous studies claiming to have found at best indirect evidence for its formation," says physicist Al Yazdani of Princeton University.
"Visualizing this crystal allows us not only to watch its formation, confirming many of its properties, but we can also study it in ways you couldn't in the past."
A crystal refers to the way atoms can be arranged in solid matter. In typical crystalline materials, the atoms are bound to one another in a way that forms a repeating pattern in space.
Wigner's groundbreaking 1934 paper proposed that electrons could form similar arrangements, aided – not hindered – by the mutual revulsion generated by the negative charge carried by all electrons.
At extremely low temperatures and at low densities, the repulsive interaction between electrons should, he theorized, see their potential energy dominate their need to zoom about, causing them to fall into crystal-like lattice arrangements.
These crystals would not behave according to classical physics, but quantum mechanics, with the bound electrons behaving not like discrete particles but an individual wave. A variety of experiments involving two-dimensional systems designed to detect the outcomes of this behavior have ponied up indirect evidence of Wigner crystals, but direct evidence has been a bit trickier to come by.
"There are literally hundreds of scientific papers that study these effects and claim that the results must be due to the Wigner crystal," Yazdani says, "but one can't be sure, because none of these experiments actually see the crystal."
With the flaws of these experiments in mind, a team led by physicists Yen-Chen Tsui, Minhao He, and Yuwen Hu of Princeton University designed an experiment that they hoped would resolve previous problems and reveal the crystal.
They used magnetic fields to induce an electron Wigner crystal in graphene, but not just any old graphene. The material had to be as pristine as possible, to eliminate any effects that might be generated by atomic imperfections.
Two sheets of graphene were prepared and arranged in a specific configuration before being cooled to just a fraction above absolute zero. A magnetic field was then applied to tune the density of the electron gas sandwiched between the layers.
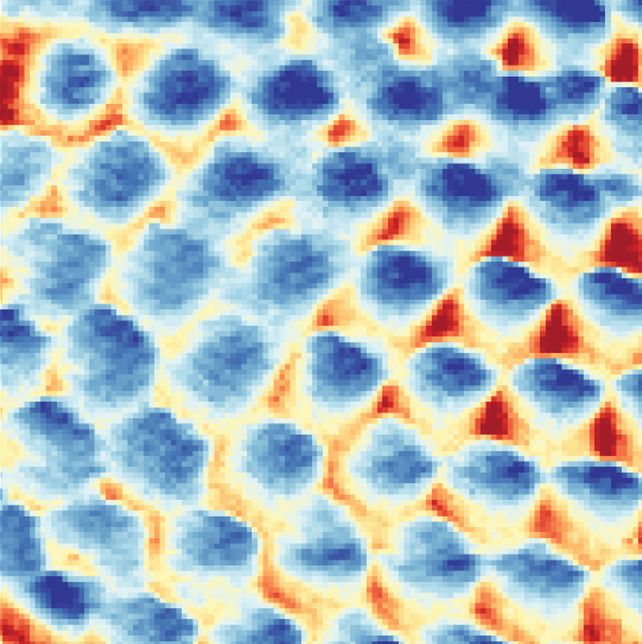
The Wigner crystal has an electron density sweet spot. If the density is too low, the electrons will push each other away and they'll just sort of zoom off. If the density is too high, the electrons will mush together into an electron liquid.
At the Goldilocks spot, the electrons will try to repel each other… but their escape will be cut off by other electrons. So they'll just sort of arrange themselves into a grid, maintaining as much equidistance between them as possible.
To measure this crystal phase, the researchers used high-resolution scanning tunneling microscopy (STM) to measure it. STM uses quantum tunneling to probe materials on an atomic scale, where optical microscopy can't quite reach.
"In our experiment, we can image the system as we tune the number of the electrons per unit area. Just by changing the density, you can initiate this phase transition and find electrons spontaneously form into an ordered crystal," Tsui explains.
"Our work provides the first direct images of this crystal. We proved the crystal is really there and we can see it."
Their measurements also confirmed models describing the lattice as triangular when confined to a 2D space, though found it can remain stable as the density is tuned to quite a large degree – contradicting previous theories that the density range must be quite small. They also found that the electrons don't occupy a single point in the lattice, but a blurry range of positions described as zero-point motion.
"Electrons, even when frozen into a Wigner crystal, should exhibit strong zero-point motion," says Yazdani. "It turns out this quantum motion covers a third of the distance between them, making the Wigner crystal a novel quantum crystal."
The findings have been published in Nature.