For the first time, an international team of physicists has successfully manipulated small numbers of light particles – known as photons – that have a strong relationship with each other.
That may sound a little obscure, but it's a fundamental breakthrough in the quantum realm that could lead to technology we currently can't even dream of. Imagine lasers, but with quantum sensitivity, for medical imaging.
"This opens the door to the manipulation of what we can call 'quantum light'," says physicist Sahand Mahmoodian from the University of Sydney.
"This fundamental science opens the pathway for advances in quantum-enhanced measurement techniques and photonic quantum computing."
While physicists are getting very good at controlling quantum entangled atoms, it's proved far more challenging to achieve the same thing with light.
In this new experiment, a team from the University of Sydney and the University of Basel in Switzerland shot both a single photon and a pair of bound photons at a quantum dot (an artificially created atom) and could measure a direct time delay between the photon on its own and the ones that were bound.
"The device we built induced such strong interactions between photons that we were able to observe the difference between one photon interacting with it compared to two," says physicist Natasha Tomm, joint lead author, from the University of Basel.
"We observed that one photon was delayed by a longer time compared to two photons. With this really strong photon-photon interaction, the two photons become entangled in the form of what is called a two-photon bound state."
They set up this bound state using stimulated emission – a phenomenon first described by Albert Einstein in 1916, and which forms the basis of modern lasers. (Fun fact: laser stands for Light Amplification by Stimulated Emission of Radiation.)
Inside a laser, an electrical current or light source is used to hype up electrons within the atoms of an optical material such as glass or crystal.
This excitement bumps the electrons up an orbit in their atom's nucleus. And when they come back down to their regular state, they emit energy in the form of photons. These are the "stimulated" emissions and this process means all the resulting photons have identical wavelengths, unlike normal white light, which is a mix of different frequencies (colors).
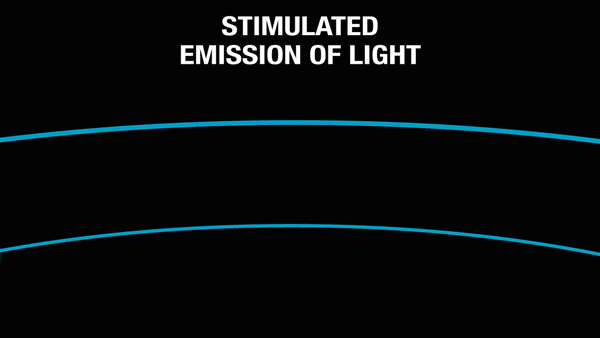
A mirror is then used to bounce the old and new photons back towards the atoms, stimulating more identical photons to be produced.
These photons move in unison, travelling with the same speed and direction, and build up until eventually they overcome the mirrors and the optical medium and blast free in a perfectly synchronized beam of light that can stay sharply focused over long distances.
All of that occurs in milliseconds when you push the button on your laser pointer (thanks, Einstein).
This type of cool interaction between light and matter is the basis for all kinds of incredible technology, such as GPS, computers, medical imaging, and global communications networks. Even LIGO, the laser interferometer gravitational-wave observatory that detected gravitational waves for the first time in 2015 is based on lasers.
But all of this technology still requires a whole lot of photons, which limits how sensitive they can be.
The new breakthrough has now achieved stimulated emission and detection for single photons, as well as small groups of photons from a single atom, leading to them becoming strongly correlated – in other words, 'quantum light'. And that's a huge step forward.
"By demonstrating that we can identify and manipulate photon-bound states, we have taken a vital first step towards harnessing quantum light for practical use," says Mahmoodian.
The next steps, she explains, are to use the approach to generate states of light that can make better quantum computers.
"This experiment is beautiful, not only because it validates a fundamental effect – stimulated emission – at its ultimate limit, but it also represents a huge technological step towards advanced applications," adds Tomm.
"We can apply the same principles to develop more-efficient devices that give us photon bound states. This is very promising for applications in a wide range of areas: from biology to advanced manufacturing and quantum information processing."
The research has been published in Nature Physics.