In a major step forward for atomic-scale quantum computing, scientists have built the first two-qubit gate between atoms in silicon, allowing qubits to communicate with each other and perform operations faster than ever before.
Since a two-qubit gate is the fundamental building block of a quantum computer, this has pretty amazing implications.
"A lot of people thought this would not be possible," said quantum physicist Michelle Simmons of the University of New South Wales (UNSW) in Australia.
Qubits are quantum bits, and they are the quantum computing equivalent of binary bits, the basic units of information. However, where bits process information in one of two states - a 1 or a 0 - a qubit can be in the state of a 1, a 0, or both simultaneously, based on their spin states.
The latter state - 1 and 0 at the same time - is known as superposition.
As we reported earlier this year, maintaining the qubits' superposition enables quantum computers to solve complex mathematical problems by running calculations based on the probability of an object's state before it is measured.
But for more efficient computations, we want qubits to be able to communicate with each other. Hence, the two-qubit gate, which a team at UNSW only managed to achieve in 2015.
The technology has come a long way. Earlier this year, researchers were able to measure accuracy of two-qubit operations.
Now, by placing two atom qubits closer together than they've ever been, and measuring and controlling their spin states in real time, a different team led by Simmons has reduced the time of a two-qubit operation to just 0.8 nanoseconds.
That's 200 times faster than any other two-qubit gate developed to date.
"Atom qubits hold the world record for the longest coherence times of a qubit in silicon with the highest fidelities," Simmons said.
"Optimising every aspect of the device design with atomic precision has now allowed us to build a really fast, highly accurate two-qubit gate, which is the fundamental building block of a scalable, silicon-based quantum computer."
It's jaw-dropping work. First, the team had to work out the optimal distance to place the two phosphorus atoms for quantum operations. That turned out to be just 13 nanometres.
Then, they had to use a scanning tunnelling microscope - an instrument designed to image surfaces on an atomic level - to place and encapsulate the atoms in the silicon with high precision, as well as the circuitry required to control and read the qubits' spin states.
Not only could the team measure changes in the qubits in real-time, they could also control how strongly the two interacted.
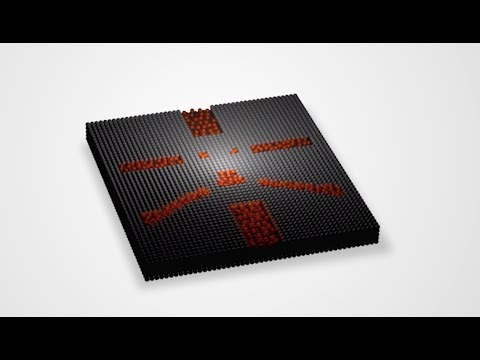
"We were able to bring the qubit's electrons closer or further apart, effectively turning on and off the interaction between them, a prerequisite for a quantum gate," said quantum physicist Yu He.
"The tight confinement of the qubit's electrons, unique to our approach, and the inherently low noise in our system enabled us to demonstrate the fastest two qubit gate in silicon to date."
It is, he added, perfectly suited to sending information between two qubits. And, when combined with a single qubit gate, it can run any algorithm you throw at it.
Theoretically, this is scalable. Developing it further is going to take a bit more time, but this achievement is a pretty important milestone that opens up the door to that scalability.
"This was one of Michelle's team's final milestones to demonstrate that they can actually make a quantum computer using atom qubits," said Emma Johnston, Science Dean of UNSW.
"Their next major goal is building a 10-qubit quantum integrated circuit - and we hope they reach that within 3-4 years."
Bring it on.
The research has been published in Nature.