Earth's solid iron core isn't all that it seems. In fact, just this year, scientists discovered that our planet's innermost orb isn't smooth, but rather textured; and stops spinning every seven decades before changing direction.
Now, in another surprising study, a team of researchers think they have figured out why Earth's solid iron core is a tad softer than expected: its atoms might move.
In Earth's inner core, some 5,100 kilometers (or 3,170 miles) beneath our feet, iron atoms are tightly packed into a hexagonal structure, compressed under enormous pressure and high temperatures.
Recent seismic observations have revealed how Earth's inner sphere exhibits some intriguing properties, more like soft metals such as lead and closer to molten iron than the solid lump we may have been picturing in our minds.
According to Youjun Zhang, a physicist at Sichuan University in China, and colleagues from the US and China who ran a series of computer simulations and lab experiments, that's because iron atoms in the inner core switch positions within their proposed hexagonal lattice structure.
Like people changing seats at a dinner table, the iron atoms migrate to neighboring positions without disturbing the underlying metallic structure of the iron, which makes the core more malleable, Zhang and colleagues suggest.
"Solid iron becomes surprisingly soft deep inside the Earth because its atoms can move much more than we ever imagined," explains Zhang. "This increased movement makes the inner core less rigid, weaker against shear forces."
Prior to this, scientists had simulated Earth's inner core using computer models that tended to capture fewer than a hundred atoms arranged in a repeating hexagonal structure.
Some researchers also suggested that melt pockets within Earth's inner core may explain some of its observed properties.
But those pockets likely got squeezed out as the core solidified, Zhang and colleagues suggest, and no theory has as yet comprehensively explained the strange suppleness of Earth's inner orb.
To expand their view of lattice dynamics, Zhang and colleagues used a supercomputer and machine learning algorithm to simulate a much larger atomic environment, of more than 10,000 atoms.
The researchers fed their model data collected from high pressure-temperature lab experiments designed to emulate conditions of Earth's inner core.
At pressures ranging from 230 to 330 GPa and temperatures just below the melting point of iron, simulations of the close-packed lattice structure suggest the iron atoms move in a pattern of collective motion "wherein one atom jumps out of its equilibrium position and pushes its neighboring atoms along."
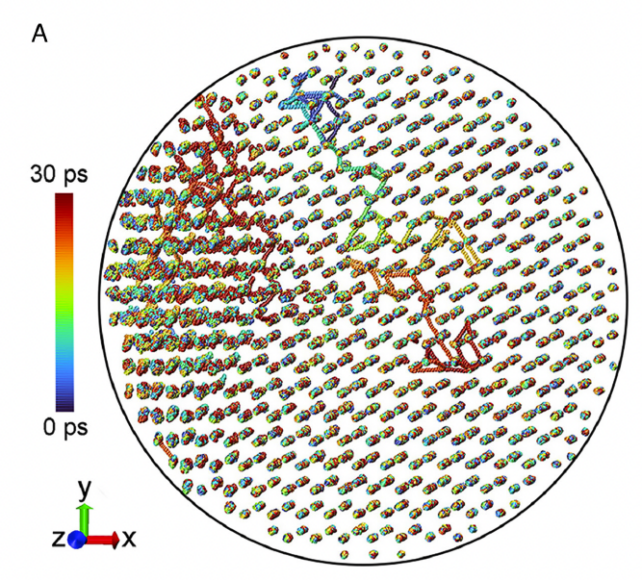
This fast diffusion occurs over picoseconds, one trillionth of a second, so the movement doesn't disrupt the lattice structure. Instead, the atoms jiggle about such that the iron core behaves like an extremely soft solid.
These results are, of course, based on theoretical calculations of a substance scientists can't sample, and can only infer its properties from afar. Bearing in mind those limitations, the findings square well with seismic observations.
"Now, we know about the fundamental mechanism that will help us with understanding the dynamic processes and evolution of the Earth's inner core," says senior author Jung-Fu Lin, a geoscientist at the University of Texas.
The study has been published in PNAS.