Despite our knack for tying knots in everything from shoelaces to laser beams, there is still a lot we humans can learn from a tiny tangle-inclined worm.
Commonly known as the California blackworm, the tiny invertebrate Lumbriculus variegatus has graciously shared a few of its secrets in a new study examining the math behind its incredible living knots.
Researchers at the Georgia Institute of Technology in the US have studied blackworms for years, intrigued by their capacity for ultrafast movement and collective behavior – namely, how they form massive knots, or "blobs," with thousands of worms that can also disperse in milliseconds.
In the new study, researchers from Georgia Tech and the Massachusetts Institute of Technology used ultrasound to shed new light on worm blobs, revealing details that could inform the design of robots with similar skills.
"We wanted to understand the exact mechanics behind how the worms change their movement dynamics to achieve tangling and ultrafast untangling," says co-author Saad Bhamla, assistant professor in the School of Chemical and Biomolecular Engineering at Georgia Tech.
"Also, these are not just typical filaments like string, ethernet cables, or spaghetti – these are living, active tangles that are out of equilibrium, which adds a fascinating layer to the question," he adds.
Wild blackworms live in North America and Eurasia, where they inhabit shallow waters at the edges of ponds, lakes, and marshes, eating dead plants and microorganisms in the mud.
Individuals range in length from about 4 to 8 centimeters (1.5 to 3 inches), but they can also intricately tangle themselves together, forming a living worm blob with up to 50,000 individuals.
Blobbing helps them survive harsh conditions, like extreme temperatures or lack of water, that would kill lone worms. A worm blob can behave as a solid or fluid, research has found, and can even demonstrate collective decision-making.
And while the worms may spend several minutes weaving together, they can separate themselves in just milliseconds.
"I was shocked when I pointed a UV light toward the worm blobs and they dispersed so explosively," says George Tech bioengineer Harry Tuazon, a graduate student in Bhamla's lab.
"But to understand this complex and mesmerizing maneuver, I started conducting experiments with only a few worms."
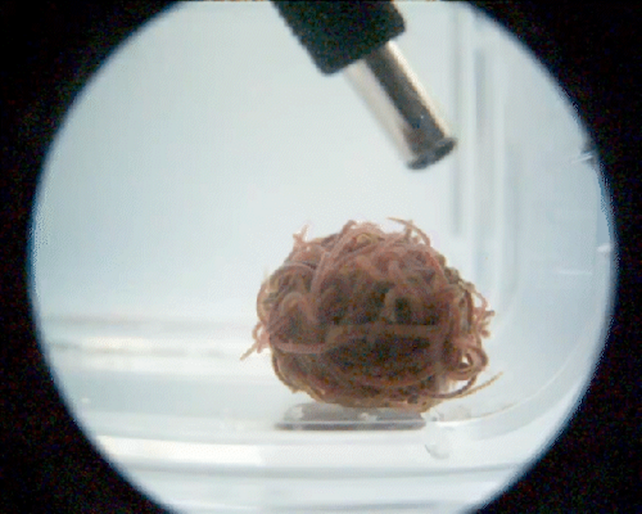
After seeing Tuazon's videos of the worms' rapid dispersal from a blob, bioengineer Vishal Patil (now at Stanford University) and colleagues leapt at the chance to team up and study them.
"Knots and tangles are a fascinating area where physics and mechanics meet some very interesting math," Patil says. "These worms seemed like a good playground to investigate topological principles in systems made up of filaments."
Patil noticed in one video a worm was moving in a figure-eight pattern, a "helical gait" that has been known in blackworms for decades. Patil, however, wondered if the move might also be part of the secret for ultrafast blob breakups.
The researchers hoped to mathematically explain a worm blob, modeling how the worms tangle and disperse, but they would need more data. And recording precise imagery of a blob's structure proved difficult.
"Capturing the inside structure of a live worm blob was a real challenge," Tuazon says. "We tried all sorts of imaging techniques for months, including X-rays, confocal microscopy, and tomography, but none of them gave us the real-time resolution we needed."
Eventually, the researchers found a technique that worked: ultrasound. They immobilized a worm blob in nontoxic jelly and used a commercial ultrasound machine to peer inside.
Bhamla, Tuazon, and other researchers at Georgia Tech analyzed the resulting ultrasound videos, then plotted some 46,000 data points to help Patil and Dunkel study the mechanics and topology of worm blobs.
They used these data to create a mathematical model for blackworm tangling and untangling, predicting each worm should intertwine with at least two others as they coalesce. It also suggested helical gaits are key to the speedy dispersals.
Visualizations of their model closely match actual videos of worms tangling and untangling, the researchers report, showing how the worms' helical motions let them quickly tangle in a way that sets up a quick-release mechanism, which relies on similar movements.
"What's striking is these tangled structures are extremely complicated," Patil says. "They are disordered and complex structures, but these living worm structures are able to manipulate these knots for crucial functions."
Studying worm knots could have many practical applications, the researchers note, such as synthetic filaments or shape-shifting robots that can change properties on demand.
"Imagine a soft, nonwoven material made of millions of stringlike filaments that can tangle and untangle on command, forming a smart adhesive bandage that shape-morphs as a wound heals, or a smart filtration material that alters pore topology to trap particles of different sizes or chemical properties," Bhamla says. "The possibilities are endless."
The study was published in Science.