For a while, in the Middle Ages, there was a real craze for trying to turn unassuming lead into pure, gleaming gold.
Perhaps those ancient alchemists should have been building a particle collider. According to a new paper, CERN's Large Hadron Collider produced about 86 billion gold nuclei from high-speed lead nuclei during the facility's second run, between 2015 and 2018.
This is not actually much gold – mere trillionths of a gram. Nor does it last very long – just fractions of a second. But what's really cool here is the way physicists quantified the gold production: by counting the number of protons accompanying neutrons involved in the lead interactions using the ALICE (A Large Ion Collider Experiment) detector's zero degree calorimeters (ZDCs).
"Thanks to the unique capabilities of the ALICE ZDCs, the present analysis is the first to systematically detect and analyze the signature of gold production at the LHC experimentally," explains physicist Uliana Dmitrieva of the ALICE collaboration at CERN.
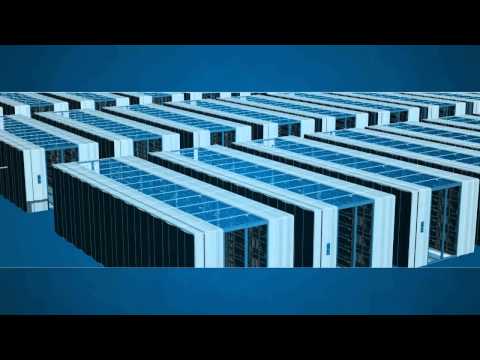
On the periodic table, lead and gold are separated by just a few spaces. Gold has 79 protons and lead has 82, so you can essentially knock a few protons (plus some neutrons) off a lead atom and end up with a gold atom.
The process is analogous to the chrysopoeia that alchemists attempted, but practically, it's not quite that simple. You need a particle collider that can accelerate particles to energies high enough to do the knocking.
In short, it's extremely energy-intensive, and requires very expensive, highly specialized equipment. If you want gold, it's probably the least efficient way to get it, in terms of effort, cost, and resources.
But lead is a popular choice for particle collider experiments, resulting in the very brief production of gold as a by-product.
The ALICE collaboration has now quantified the gold production not from lead nuclei smacking into each other, but from near misses as they fly around at 99.999993 percent of the speed of light in the Large Hadron Collider.
At these speeds, the lead nucleus, with its 82 charged protons, flattens the electromagnetic field in the collider perpendicular to the direction in which it is traveling, generating a pulse of photons when two lead nuclei pass each other closely enough.
An interaction with a photon can then wobble the interior structure of a lead nucleus, causing it to eject neutrons and protons.
It's not just gold that emerges from this process. The removal of nucleons can produce a thallium nucleus with 123 neutrons and 81 protons; or a mercury nucleus with 121 neutrons and 80 protons.
Using ALICE's ZDCs to count loose neutrons with one, two, or three protons, the collaboration quantified the production of all three elements during the same run of the Large Hadron Collider.
Thallium and mercury are produced in far greater quantities than gold, but the latter is currently produced at a maximum rate of around 89,000 nuclei per second, from lead-lead collisions near the ALICE collision point in the collider.
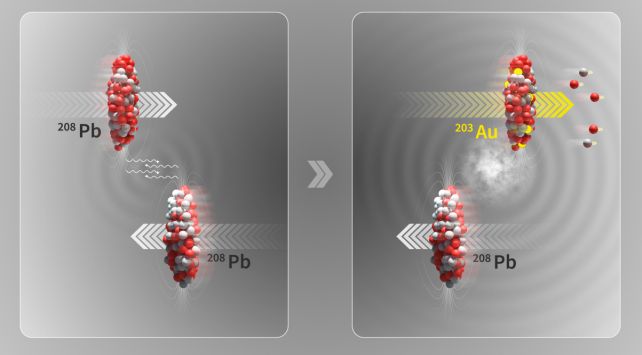
For the particle accelerator's second run, the amount of gold produced was minuscule – just 29 picograms, or trillionths of a gram. That's the scale on which bacteria are measured. There are sextillions of atoms in just a single gram of gold.
In addition, the high-speed gold nuclei then smash against the sides of the Large Hadron Collider and disintegrate in a shower of protons, neutrons, and electrons almost as soon as they form. The medieval alchemists would have been deeply disappointed.
We're not, though. This is really fascinating science. Not only can scientists hurl atoms at each other at almost light speed, they can then determine the changes those atoms undergo as a result of the hurling. That's far beyond the wildest dreams of our medieval forebears.
"It is impressive to see that our detectors can handle head-on collisions producing thousands of particles," says particle physicist Marco van Leeuwen, of Utrecht University, spokesperson for the ALICE collaboration, "while also being sensitive to collisions where only a few particles are produced at a time, enabling the study of rare electromagnetic 'nuclear transmutation' processes."
The findings have been published in Physical Review C.