Somewhere out in the depths of the Milky Way galaxy, something stirs. Powerful forces whip charged particles into an energetic frenzy of cosmic rays, launching them at velocities that near the speed of light.
We may finally be close to pinning down their origins.
A reanalysis of 10 years of data collected by the the IceCube Neutrino Observatory in Antarctica has returned the most solid evidence to date of neutrino emissions from the center of our galaxy that point to long-sought sources of cosmic rays.
"Confirming the existence of this long-sought signal paves the way for the future of astroparticle physics in our galaxy," writes physicist Luigi Antonio Fusco from Italy's Università degli Studi di Salerno, in a Perspective about the discovery published in Science.
It also provides us with the first neutrino map of our Milky Way, and a new way of viewing our galactic plane.
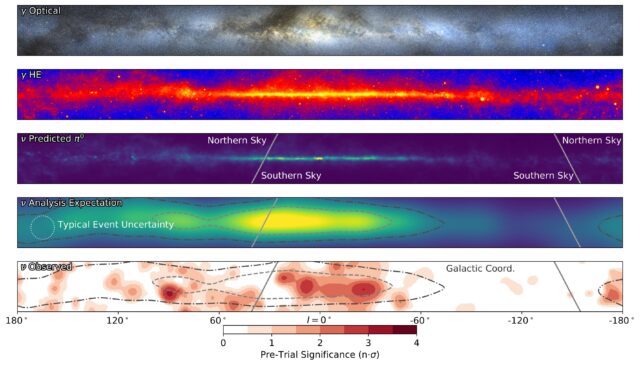
Poor little old Earth is peppered constantly by cosmic bullets of protons and charged atomic cores shot from somewhere out in the Milky Way, accelerated to insane speeds by powerful fields. Tracing many back to their source is literally far from straight forward.
"Cosmic ray particles that are electrically charged aren't suitable for studying cosmic ray sources," Lindsey Bignell, an experimental particle physicist from the Australian National University who wasn't involved in the study, told ScienceAlert.
"They're influenced by magnetic fields so don't travel in a straight line from their source to us."
There is one way we just might be able to pick up on their trail. We can search for signs of carnage left by the chunky cosmic particles as they careen into gas and dust in the interstellar medium.
One product of these atomic smash-ups is a pairing of a quark and an antiquark, known as a pion. Neutral versions of these short-lived pairings evaporate into a flash of gamma rays as they quickly decay, which we can easily see from afar.
Catching the high energy photons has delivered a rough map of where in the galaxy cosmic rays could be born, though it's hard to rule out other sources of gamma rays, such as energetic electrons also smashing into things.
Where neutral pions emit gamma rays when they decay, the breakdown of charged pions creates something a little different – a highly energetic electron neutrino.
Neutrinos shimmer on the edge of existence, giving them the quaint title of 'ghost particle'. With barely any mass and no charge to make their presence known they can fly on a straight path through the Universe close to the speed of light, stopping only when they happen to meet an atom's nucleus head-on.
Spotting such collisions here on Earth takes incredible patience, but isolated facilities like IceCube have amassed years of observations. There's just one problem – neutrinos come from a variety of other sources, such as our very own atmosphere.
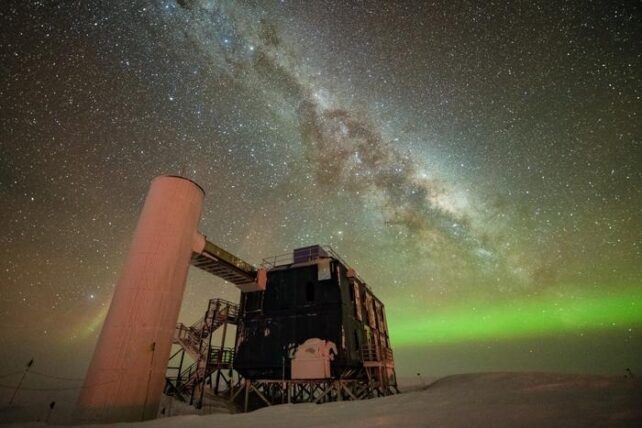
"To see the astrophysical neutrinos from the galactic plane reported in this study, the authors first needed to overcome a background of atmospheric neutrinos," Bignell told ScienceAlert.
"The authors reduced this background by excluding the main type of atmospheric neutrino, muon neutrinos. Muon neutrinos generate distinctive straight tracks, which allow easy reconstruction of the particle direction necessary for neutrino astronomy."
Past attempts to sift out these straight tracks from those produced by neutrinos of astrophysical origins have struggled to deliver anything close to statistically significant.
So researchers from the IceCube Collaboration attempted something a little different, handing the task of learning the features of straight tracks produced by muon neutrinos from our own atmosphere and the 'electron' style neutrinos produced by distant cosmic ray collisions to a computer.
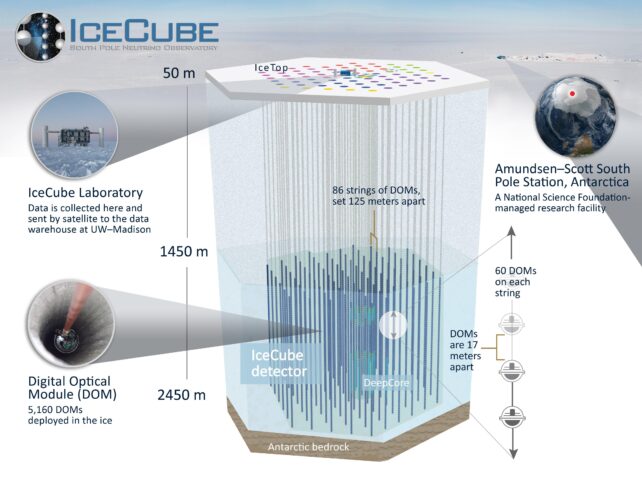
"The authors used machine learning to improve upon their previous data analysis methods, and this allowed them to include 20 times more events in their dataset, with better directional information," says Bignell.
The new analysis of the IceCube data successfully picked out a diffuse emission of neutrinos shining from the center of the Milky Way with a statistical significance of around 4.5 sigma. With a few tweaks and a little more data, it might even be possible to resolve details in that glow, giving us a new kind of cosmic vision.
In spite of falling a little short of the '5 sigma' physicists seek in order to confidently accept a finding as reliable (well, as reliable as science gets), the results represent a milestone in using neutrinos as a means of mapping our Universe.
Where visible light was once the only way we could study the heavens, modern science now has a litany of tools at its disposal, from the quiet of low energy radio emissions to high energy photons to the very rumble of spacetime.
We're on the cusp of seeing reality in a whole new way, using particles that are barely even there to see things we once barely even imagined.
This research was published in Science.