The very first stars might have appeared when the Universe was only 100 million years old, or less than 1 percent of its current age. Since then, the rapid expansion of space has stretched their light into oblivion, leaving us to seek clues about their existence in cosmic sources closer to home.
By analyzing the light emerging from clouds around a distant quasar, researchers from Japan, Australia, and the United States found a "distinctive blend of heavy elements" could have come from just one source: the colossal supernova of a first-generation star.
All of the stars we can observe are classified as either Population I or Population II, depending on their age. Population I stars are younger and contain more heavy elements, while Population II stars are older with fewer heavy elements.
The very first stars – described as Population III – are older still, their existence coinciding with cosmic distances that put them well out of sight of even our best technologies. For now, we can only theorize what they might have looked like.
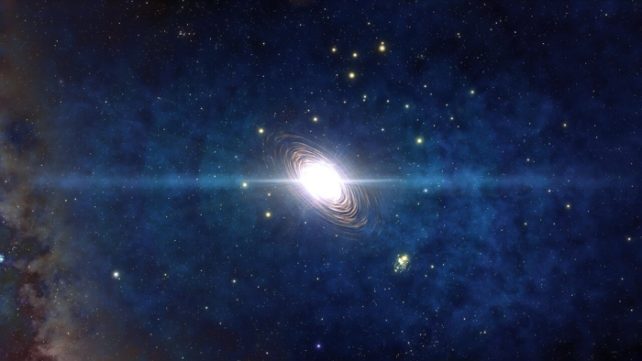
Scientists think those earliest stars were super hot, bright, and massive, maybe hundreds of times the mass of our Sun.
Without a history of powerful cosmic events to generate elements heavier than lithium, Population III stars would consist entirely of the simplest of gases. Back then, the only materials available in the Universe were hydrogen, helium, and a little lithium, found in primordial gas left over from the Big Bang. Only once the first stars themselves collapsed in heated violence could heavier elements emerge.
Those first stars likely concluded their lives with pair-instability supernovae, a theoretical type of super-supernova only possible in such massive stars. Unlike other supernovae, this would leave behind no stellar remnants like a neutron star or black hole, instead blasting everything outward in an ever-expanding cloud.
That blast might have seeded ancient interstellar space with the heavy elements needed for the formation of rocky worlds like our own — thus enabling life as we know it — so the net effect is positive.
For astronomers on Earth now hoping to learn about Population III stars, however, the light from those ancient mega-explosions has faded into the distance, leaving little more than a diffuse cloud containing a complex mix of elements.
Given time, that mix of material could itself collapse into something new. To find signs of such a concentration of star dust, the authors of the new study used near-infrared spectrograph data from one of the most distant-known quasars — a type of active galactic nucleus, or the extremely luminous center of a young galaxy.
This quasar's light had been speeding through space for 13.1 billion years before it reached Earth, the researchers note, which means we're seeing the quasar as it looked when the Universe was only 700 million years old.
A spectrograph is an instrument that captures and splits incoming light, in this case from a celestial object, into its component wavelengths. This can reveal which elements are present in a faraway object, although gleaning that information isn't always easy.
The brightness of lines in astronomical spectra can hinge on factors other than the abundance of an element, the authors point out, which may complicate efforts to identify specific elements.
Yet two of the study's authors – astronomers Yuzuru Yoshii and Hiroaki Sameshima, both from the University of Tokyo – had already developed a trick to get around this problem.
Their method, which involves using wavelength intensity to estimate the prevalence of elements, allowed the research team to analyze the composition of clouds around this quasar.
The analysis revealed a strangely low ratio of magnesium to iron in the clouds, which had 10 times more iron than magnesium compared with our Sun. That was a clue, the researchers say, suggesting this was material from the cataclysmic explosion of a first-generation star.
"It was obvious to me that the supernova candidate for this would be a pair-instability supernova of a Population III star, in which the entire star explodes without leaving any remnant behind," says co-author Yuzuru Yoshii, an astronomer at the University of Tokyo.
"I was delighted and somewhat surprised to find that a pair-instability supernova of a star with a mass about 300 times that of the Sun provides a ratio of magnesium to iron that agrees with the low value we derived for the quasar."
At least one other potential trace of a Population III star was reported in 2014, Yoshi and his colleagues note, but they argue this new finding is the first to provide such strong evidence.
If they're right about what they found, this research could go a long way in revealing how matter evolved during the history of the Universe. But to be certain, they add, more observations will be needed to check for similar traits in other celestial objects.
Those observations might not all need to come from such faraway quasars. Even if there are no more Population III stars left in the Universe, the longevity of their supernova remnants means evidence could be hiding almost anywhere – including the local Universe around us.
"We now know what to look for; we have a pathway," says co-author Timothy Beers, an astronomer at the University of Notre Dame.
"If this happened locally in the very early Universe, which it should have done, then we would expect to find evidence for it."
The findings were published in The Astrophysical Journal.