The Universe should be humming.
Every supernova, every merger between neutron stars or black holes, even rapidly spinning lone neutron stars, could or should be a source of gravitational waves.
Even the rapid inflation of space following the Big Bang 13.8 billion years ago should have produced its own cascade of gravitational waves.
Like a rock thrown in a pond, these massive events should send ripples reverberating through the very fabric of space-time – faint expansions and contractions of space that could be detectable to us as discrepancies in what should be precisely timed signals.
Collectively, this mix of signals combines to form a random or 'stochastic' buzz known as the gravitational wave background, and it's one of possibly the most highly-sought detections in gravitational wave astronomy.
The new frontier in space exploration
It's thought – just as the discovery of the cosmic microwave background did before it (and continues to do) – that finding the gravitational wave background will blow our understanding of the Universe and its evolution wide open.
"Detecting a stochastic background of gravitational radiation can provide a wealth of information about astrophysical source populations and processes in the very early Universe, which are not accessible by any other means," explains theoretical physicist Susan Scott of the Australian National University and the ARC Centre of Excellence for Gravitational Wave Discovery.
"For example, electromagnetic radiation does not provide a picture of the Universe any earlier than the time of last scattering (about 400,000 years after the Big Bang). Gravitational waves, however, can give us information all the way back to the onset of inflation, just ∼10-32 seconds after the Big Bang."
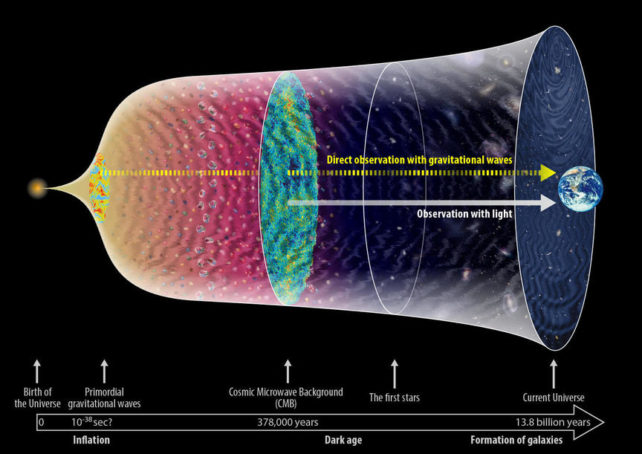
To understand the importance of the gravitational wave background, we ought to talk a little bit about another relic of the Big Bang: the cosmic microwave background, or CMB.
Moments after our Universe started ticking and space began to cool, the bubbling foam that was everything congealed into an opaque soup of subatomic particles in the form of ionized plasma.
Any radiation that emerged with it was scattered, preventing it from making it any great distance. It wasn't until these subatomic particles recombined into atoms, an era known as the Epoch of Recombination, that light could freely move through the Universe and on down through the eons.
The first flash of light burst through space around 380,000 years after the Big Bang, and, as the Universe grew and grew in the following billions of years, this light got dragged into every corner. It's still all around us today. This radiation is extremely faint but detectable, particularly in microwave wavelengths. This is the CMB, the first light in the Universe.
The irregularities in this light, referred to as anisotropies, were caused by small temperature fluctuations represented by that first light. It's difficult to overstate how phenomenal its discovery was: the CMB is one of the only probes we have of the state of the early Universe.
The discovery of the gravitational wave background would be a magnificent replication of this achievement.
"We expect the detection and analysis of the gravitational wave background to revolutionize our understanding of the Universe," Scott says, "in the same way pioneered by the observation of the cosmic microwave background and its anisotropies."
The buzz beyond the boom-crash
The first detection of gravitational waves was made just a short time ago, in 2015.
Two black holes that collided roughly 1.4 billion years ago sent ripples propagating at light-speed; on Earth, these expansions and contractions of space-time very faintly triggered an instrument designed and refined for decades, waiting to detect just such an event.
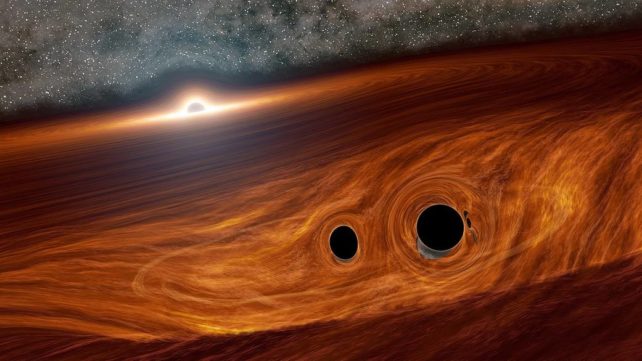
It was a monumental detection for several reasons. It gave us direct confirmation, for the first time, of the existence of black holes.
It confirmed a prediction made by the General Theory of Relativity 100 years earlier that gravitational waves are real.
And it meant that this tool, the gravitational wave interferometer, that scientists had been working on for years would revolutionize our understanding of black holes.
And it has. The LIGO and Virgo interferometers have detected nearly 100 gravitational wave events to date: those strong enough to produce a marked signal in the data.
These interferometers use lasers shining down special tunnels several kilometers long. These lasers are affected by the stretching and squeezing of space-time produced by gravitational waves, generating an interference pattern from which scientists can infer the properties of the compact objects generating the signals.
But the gravitational wave background is a different beast.
"An astrophysical background is produced by the confused noise of many weak, independent, and unresolved astrophysical sources," Scott says.
"Our ground-based gravitational wave detectors LIGO and Virgo have already detected gravitational waves from tens of individual mergers of a pair of black holes, but the astrophysical background from stellar mass binary black hole mergers is expected to be a key source of the GWB for this current generation of detectors. We know that there are a large number of these mergers which cannot be resolved individually, and together they produce a hum of random noise in the detectors."
The rate at which binary black holes collide in the Universe is unknown, but the rate at which we can detect them gives us a baseline from which we can make an estimate.
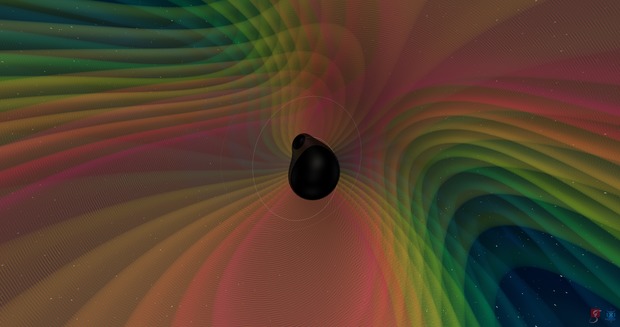
Scientists believe it's between around one merger per minute, and several per hour, with the detectable signal of each lasting just a fraction of a second. These individual, random signals would probably be too faint to detect but would combine to create a staticky background noise; astrophysicists compare it to the sound of popcorn popping.
This would be the source of a stochastic gravitational wave signal we could expect to find with instruments like the LIGO and Virgo interferometers. These instruments are currently undergoing maintenance and preparation and will be joined by a third observatory, KAGRA in Japan, in a new observing run in March 2023. A detection of the popcorn GWB by this collaboration is not out of the question.
These are not the only tools in the gravitational wave kit, though. And other tools will be able to detect other sources of the gravitational wave background. One such tool, still 15 years away, is the Laser Interferometer Space Antenna (LISA), set to be launched in 2037.
It's based on the same technology as LIGO and Virgo, but with "arms" that are 2.5 million kilometers long. It will operate in a much lower-frequency regime than LIGO and Virgo and will therefore detect different kinds of gravitational wave events.
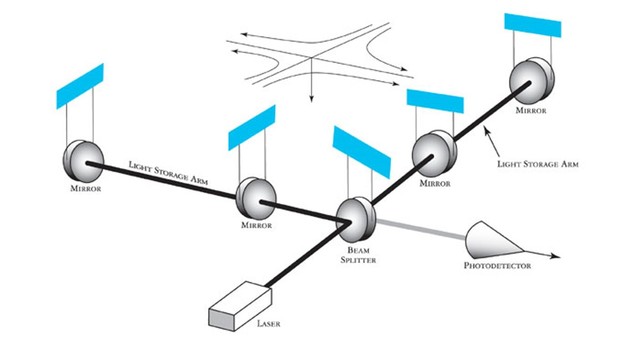
"The GWB is not always popcorn-like," Scott tells ScienceAlert.
"It can also consist of individual deterministic signals which overlap in time producing a confusion noise, similar to the background conversations at a party. An example of confusion noise is the gravitational radiation produced by the galactic population of compact white dwarf binaries. This will be an important source of confusion noise for LISA. In this case, the stochastic signal is so strong that it becomes a foreground, acting as an additional source of noise when trying to detect other weak gravitational wave signals in the same frequency band."
LISA could theoretically also detect cosmological sources of the gravitational wave background, such as cosmic inflation just after the Big Bang or cosmic strings – theoretical cracks in the Universe that could have formed at the end of inflation, losing energy via gravitational waves.
Timing the pulse of the cosmos
There's also one huge, galactic-scale gravitational wave observatory that scientists have been studying to look for hints of the gravitational wave background: pulsar timing arrays. Pulsars are a type of neutron star, the remains of once-massive stars that have died in a spectacular supernova, leaving just a dense core behind.
Pulsars rotate in such a way that beams of radio emission from their poles sweep past Earth, like a cosmic lighthouse; some of them do so at incredibly precise intervals, which is useful for a range of applications, such as navigation.
But the stretching and squeezing of space-time should, theoretically, produce tiny irregularities in the timing of pulsar flashes.
One pulsar displaying slight inconsistencies in timing might not mean much, but if a bunch of pulsars showed correlated timing inconsistencies, that might be indicative of gravitational waves produced by inspiralling supermassive black holes.
Scientists have found tantalizing hints of this source of the gravitational wave background in pulsar timing arrays, but we don't yet have enough data to determine if that is the case.
We're standing so enticingly close to a detection of the gravitational wave background: the astrophysical background, revealing the behavior of black holes throughout the Universe; and the cosmological background – the quantum fluctuations seen in the CMB, inflation, the Big Bang itself.
This, Scott says, is the white whale: the one we'll only see after the difficult work of teasing apart the background into the discrete sources that make up the noisy whole.
"While we look forward to a wealth of information to come from the detection of an astrophysically produced background, the observation of gravitational waves from the Big Bang is really the ultimate goal of gravitational wave astronomy," she says.
"By removing this binary black hole foreground, the proposed third generation ground-based detectors, such as the Einstein Telescope and Cosmic Explorer, could be sensitive to a cosmologically produced background with 5 years of observations, thereby entering the realm where important cosmological observations can be made."