Nuclear fission is the splitting of an atom's nucleus to create two (or more) lighter elements.
Though it can occasionally occur spontaneously in isotopes of some heavy elements, such as thorium and uranium, it is usually triggered by a neutron impacting the nucleus with the right amount of force.
The sudden overcrowding makes the clump of protons and neutrons unstable and prone to breaking apart, leaving not just smaller nuclei – or fissile products – but also ejecting more free neutrons, along with a burst of high-energy photons in the form of gamma radiation.
The energy released from this separation of nuclear particles has been used as a source of power since the mid 20th century.
While the energy production process doesn't release the same troublesome greenhouse gasses as fossil fuel burning, concerns over meltdown risks, long-term hazardous waste, and costs mean the atomic future many dreamed of in the past might not be easily achievable.
How is nuclear fission used to generate nuclear power?
Experiments in the 1930s involving the bombardment of atoms with nuclear particles led to models of fission that promised a significant amount of energy could be released from the right isotopes of heavy elements such as uranium.
Theory predicted uranium 235 was much more likely to undergo fission compared to other isotopes, especially if the neutrons striking its nucleus were moving at a relatively slow speed.
The release of additional neutrons from the fission process could cause other nearby atoms of U-235 to also break apart. For this chain reaction to occur, there needs to be a relatively high density of U-235 squeezed together – what's referred to as the material's 'critical mass'.
By the end of the 1930s, physicists had come up with methods for slowing neutrons enough for capture and enriching mixtures of uranium isotopes from natural resources to form critical masses of U-235. They also came up with a way to control the chain reaction to ensure the exponential production of neutrons didn't get out of control, in which case the process could turn explosive.
Over the following decade, technological advances in nuclear fission would be applied to the production of new classes of super weapons. It was only in the wake of the Second World War that engineers turned their attention back to the possibility that the nuclear fission process could be applied to the sustained generation of heat for generating electricity.
Just as the steam produced by combusting fossil fuels in a boiler turns a turbine linked to an electric generator, steam from a 'nuclear boiler' could also be harnessed to generate power.
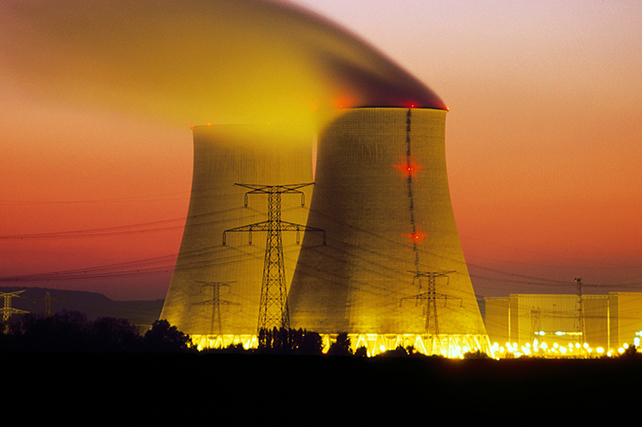
Advances in technology have continued to improve efficiency and safety over time, in some cases ditching moderators slowing down neutrons to allow fissile material to capture 'faster' particles. Today, there are around 440 nuclear power plants in operation around the globe, with nearly 100 in the United States alone. Combined, these plants produce around 10 percent of the world's electricity, down 7 percent from its peak in 1993.
In an age where the production of roughly 60 percent of the world's electricity churns out greenhouse gasses at a rate that threatens catastrophic global warming, nuclear power presents a comparatively cleaner alternative.
But there are costs that may limit how much we ought to turn to nuclear energy for salvation from the climate crisis.
What's the problem with nuclear energy?
When it comes to finding cost-effective, low-emission power alternatives to fossil fuels, we could do worse than nuclear energy. Importantly, we could also do better, with renewable energy technologies such as solar and wind that are becoming cheaper every year.
Nuclear power's challenges fall into three categories – waste, risk, and cost. Here's some examples of each.
Waste
One of the biggest public concerns over nuclear power in recent decades has been over what to do with the uranium fuel once it's so choked up with fissile products that it's no longer efficient at producing energy.
This high-level waste contains isotopes that can take thousands of years to drop in radioactivity to a level roughly matching that of the ore it came from. Right now, more than a quarter of a million metric tonnes of highly radioactive waste is in storage around the globe, waiting for disposal or reprocessing.
Is this bad? Though stored nuclear waste doesn't necessarily pose any immediate threat if it's well contained, questions over long-term management and the possibility of mishandling and mishaps make the storage of a growing pile of nuclear waste a controversial issue.
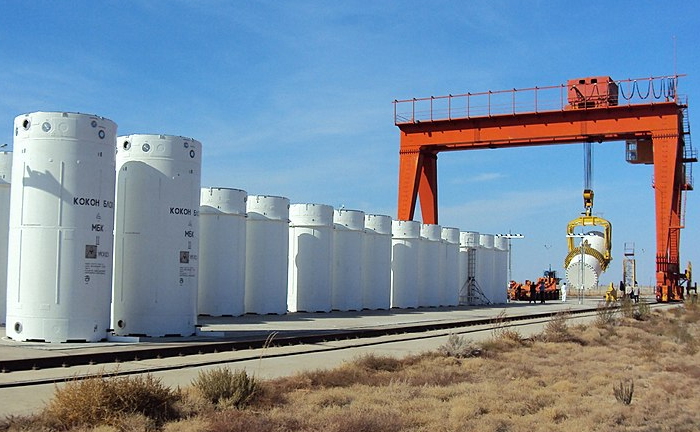
Carbon is also a waste product to consider. While the process of fission and conversion of nuclear energy into electricity is relatively free of carbon emissions, the gross carbon budget for mining and processing the ore required for fission and the construction of a concrete power plant isn't zero.
Over its lifetime, a new nuclear power plant could be responsible for emitting the equivalent of roughly 4 grams of CO2 for every kilowatt hour of electricity produced. Some estimates put the output much higher, at anywhere from 10 grams of CO2 to 130 grams in some cases.
That said, replacing coal-burning power stations with nuclear ones could save the atmosphere upwards of millions of tonnes of CO2 each year, not to mention particulates and other pollutants. By the same reasoning, clean renewables such as wind turbines and solar panels also won't have zero emissions by virtue of their manufacture and installation. The carbon footprints for solar and wind farms are more or less comparable with the lower end for nuclear.
Taken altogether, power from nuclear energy is (at best) about as carbon-free as that from solar and wind, albeit with an unpopular waste problem that few people want in their backyard.
Risk
It's been more than three decades since Soviet-era Ukraine gave the world a taste of what a worst-case scenario might look like for a nuclear accident. Following a meltdown during a technical test in 1986, the Chernobyl Nuclear Power Plant collapsed into a radioactive ruin amid a landscape poisoned by its fallout.
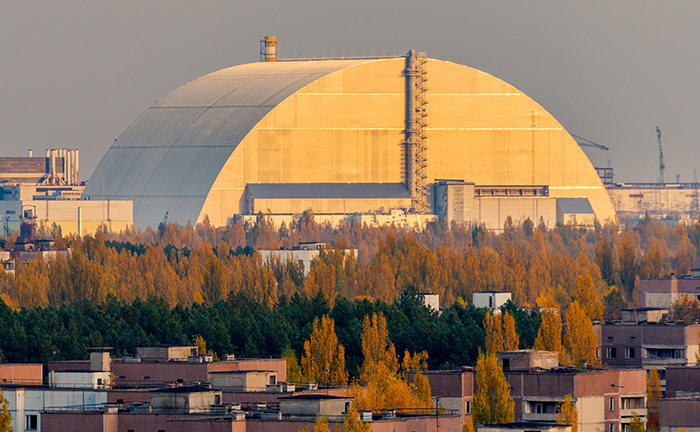
In 2011, Japan's Fukushima nuclear reactor also went into a meltdown after it was shaken by an earthquake.
Devastating events such as these are uncommon enough to be worthy of shocking headlines. Yet some estimates suggest such meltdowns could occur once every 10 to 20 years, risking the spread of radioactive material across hundreds or even thousands of kilometers of landscape.
How bad could this be? It's hard to say, depending on a wide variety of factors to do with population densities, extent of exposure, and concentrations of isotopes. According to the World Health Organization, "the displaced Fukushima population is suffering from psycho-social and mental health impact following relocation, ruptured social links of people who lost homes and employment, disconnected family ties and stigmatization".
In other words, it's not just a risk of radioactivity we'd need to be worried about.
Still, being so accustomed to the health impact of fossil fuel combustion, we give little thought to the health impact of particulates puffed out by burning coal. Which itself isn't exactly free of radioactive material either.
Cost
To compare costs of power generation, researchers use what's known as the levelized cost of energy, or LCOE. This is a measure of average net cost of generation projected over a site's lifetime.
This figure will depend on a wide range of things to do with location and fluctuations in resources. But it's still possible to get a general sense of LCOE around the world for comparing technologies.
According to the World Nuclear Industry's Status Report for 2020, the LCOE for nuclear power jumped by 26 percent over the decade between 2009 and 2019, to US$155 per megawatt hour. At the same time, coal fell by 2 percent, to US$109.
Solar photovoltaics, on the other hand, plummeted by nearly 90 percent to just US$41. Wind also fell to roughly the same cost.
Can nuclear fission power plants save the world?
Of course, new technology can always make a difference. Finding better ways to trap nuclear waste could make it safer, or at least give the public confidence that it'll be less of a threat in the future. Alternatives to uranium isotopes could take the anxiety out of meltdowns and the potential for weaponizing nuclear programs. Changing technologies could affect the scale of reactors, or even improve their LCOE altogether.
But it's likely to be too little too late.
An analysis of adoption of nuclear and renewable power generation across more than a hundred countries over the past 25 years found nuclear power just hasn't achieved the same results in carbon reduction as renewables.
What's more, investing in nuclear energy is a sunk cost that makes it harder to jump tracks towards a renewables future later.
None of this is to say nuclear power has no place in future energy production. Space exploration, for example, could benefit from advances in nuclear fission technology. Beyond energy production, the production of specific isotopes for medicine and research, all through the use of fission, is an invaluable industry.
It might not save us from the climate crisis, but the nuclear age provides other technological benefits that will be with us for a long time to come.
All Explainers are determined by fact checkers to be correct and relevant at the time of publishing. Text and images may be altered, removed, or added to as an editorial decision to keep information current.